Research Highlights
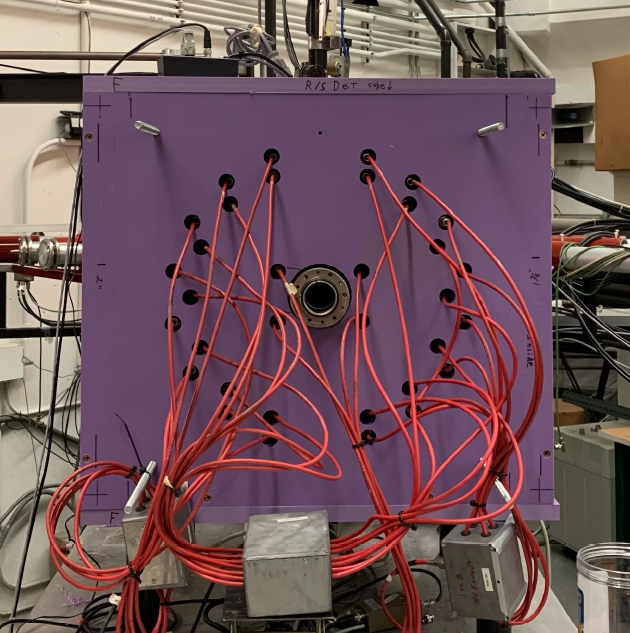
Getting the HeBGBs at the Edwards Accelerator Laboratory
Nuclear reactions involving the capture of a helium nucleus and emission of a neutron, known as (α,n) reactions, are nearly ubiqutious in nuclear astrophysics and nuclear applications. Such reactions are responsible for the synthesis of many nuclei heavier than zinc, impact long-term nuclear fuel storage, and can be used to synthesize medical isotopes. Measuring (α,n) reaction probabilities, known as cross sections, is very challenging due to the elusive nature of neutrons and special experimental designs are required. Graduate research group member Kristyn Brandenburg designed, built, and commissioned the 3HeBF3 Giant Barrel (HeBGB) detector, which is tailor-made to measure (α,n) reactions at energies of interest for astrophysics and applications. By achieving a near-constant detection efficiency over a large range of neutron energies, HeBGB overcomes a major limitation of previous generations of similar detectors. HeBGB has already been used to gather important data for both astrophysics and applications and publications on these measurements are forthcoming. The full work, published in the the Journal of Instrumentation, can be accessed here.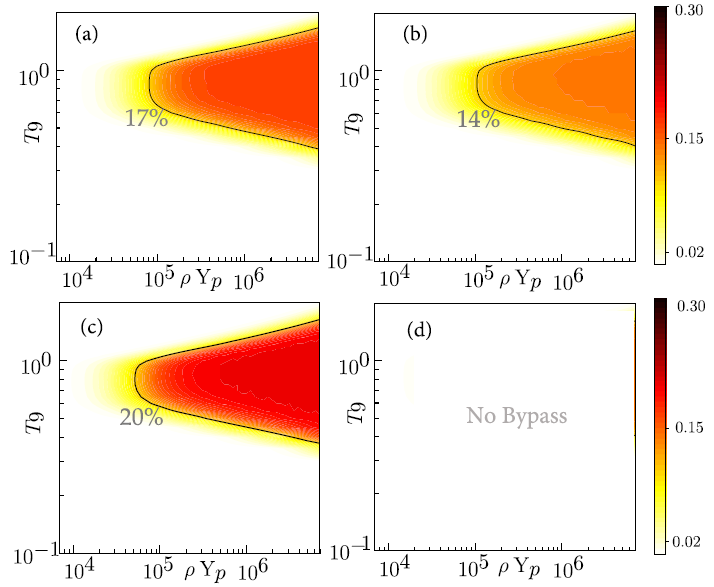
Establishing the 56Ni Bypass in the RP-Process
X-ray bursts are bright flashes from neutron stars, releasing in about a minute the same amount of energy that the Sun releases in a day. A short time after their discovery in the mid-70s, it was known that the bursts are thermonuclear explosions, powered by a sequence of hydrogen- and helium-burning reactions (now known as the rp-process) in neutron star envelopes. For decades it has been known that the characteristic peak in the light-output (known as the light-curve) is caused by the nuclear reaction sequence stalling at 56Ni, which cannot burn helium, has a low probability of burning hydrogen, and has a long decay lifetime. However, for 20 years it has been suspected that the rp-process may have a way around 56Ni, at least some of the time. The key uncertainty was how often the nucleus 57Zn emitted a proton after β-decaying. If it was 100% of the time, then there was no bypass. Postdoctoral research group member Mansi Saxena led a measurement of the 57Zn β-delayed proton-emission branching at the National Superconducting Cyclotron Laboratory. Mansi established that there is indeed a bypass of 56Ni in the rp-process, resolving this two-decade old mystery. The full work, published in Physics Letters B, can be accessed here.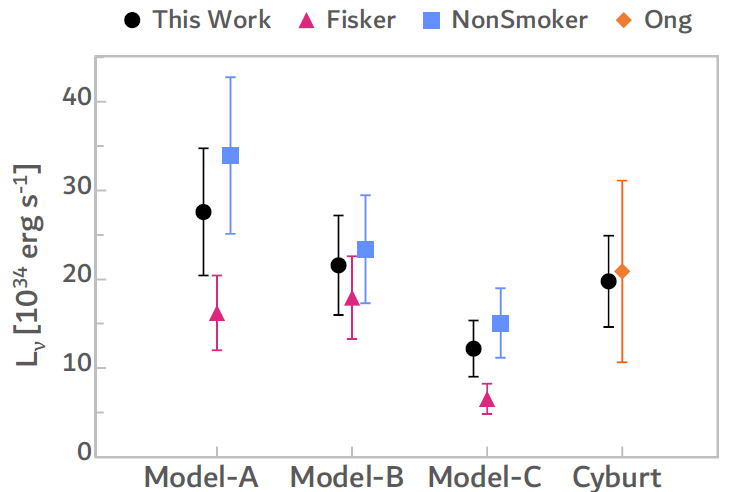
Refined 61Zn Mass Refines Urca Cooling Luminosities
Neutron stars that accrete matter siphoned from the envelope of a companion star develop rich outer layers, comprised of hundreds of nuclear species. Some nuclei are more influential than others. In particular, nuclei with mass-number (sum of protons + neutrons) equal to 61 are responsible for a significant neutrino luminosity from a process known as urca cooling. Therefore determining the production of mass 61 nuclei is of critical importance. The nuclear reaction 61Zn(p,γ) has a particularly large influence on the mass 61 abundance on neutron star surfaces and thereby in the outer layers. I worked with my collaborators to measure the mass of 61Zn to high precision, update theoretical estimates of nuclear structure needed to calculate the 61Zn(p,γ) reaction rate, implement the rate into calculations of mass 61 production on neutron star surfaces, and propagate the results into urca neutrino luminosities from mass 61 nuclei in neutron stars. Along with improving the accuracy of these neutrino luminosities, we improved the precision by a factor of two. The full work, published in Physical Review C, can be accessed here.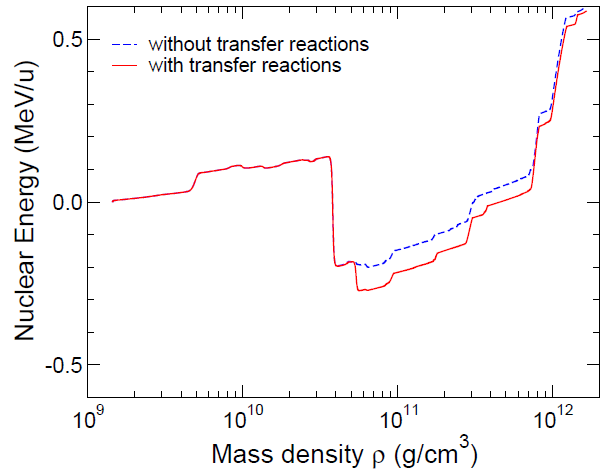
New Impact of Neutrons in Neutron Stars
Contrary to the name, a neutron star is not just a ball of neutrons. The outer 10% or so is comprised of atomic nuclei. These nuclei interact with each other and the environment to modify the thermal and compositional structure of the neutron star outer layers. A new type of nuclear reaction was recently proposed, where neutrons can hop from within one nucleus to another in a process called neutron-transfer. This can be of particular relevance in accreting neutron stars, which siphon material onto the surface, burying nuclei existing in the neutron star outer layers and further driving nuclear reactions. I worked with my colleagues to implement neutron transfer for the first time in a nuclear reaction network that models reactions in the outer layers of accreting neutron stars. We found that including these reactions modifies the structure of the neutron star outer layers, but the impacts were less significant than anticipated. The full work, published in the Astrophysical Journal, can be accessed here.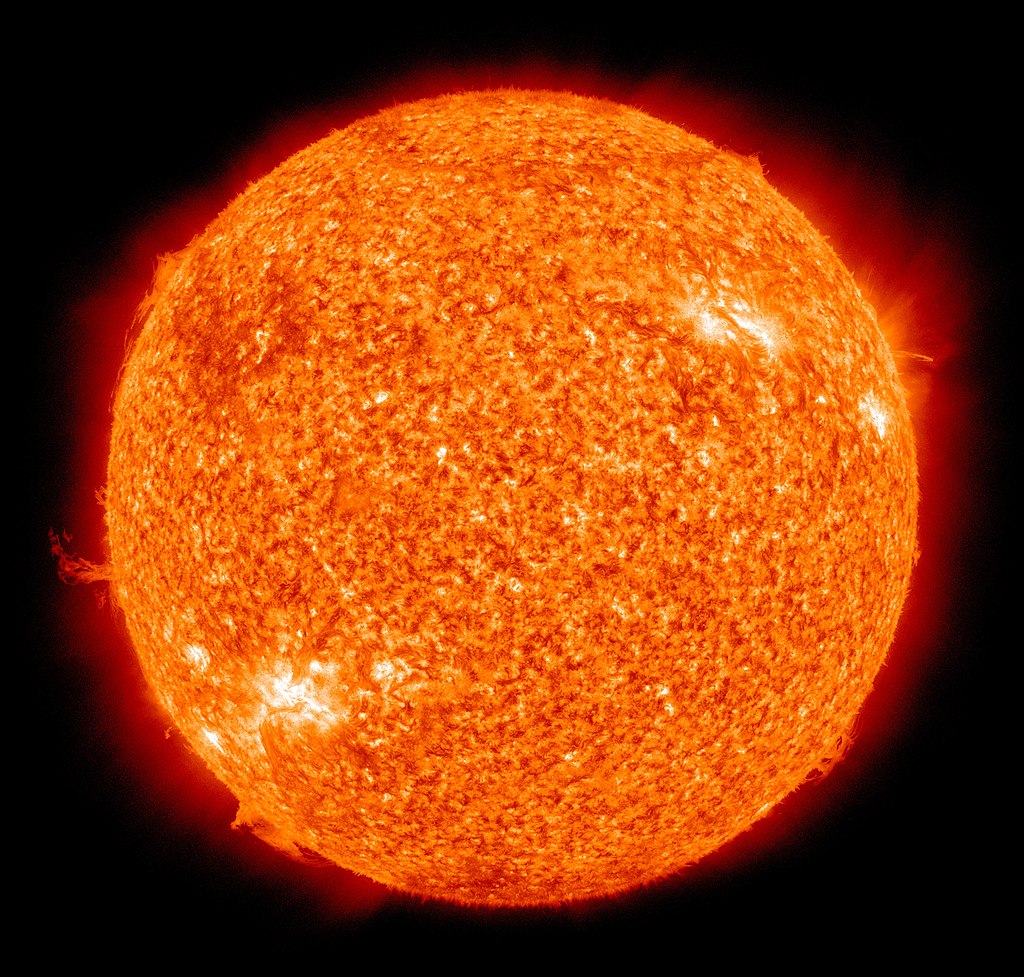
Burning Questions About Stars
The study of stellar burning began roughly a century ago, when Eddington posited in 1920 that the Sun is powered by the conversion of hydrogen into helium. Since that time, a coherent picture has emerged for nuclear reaction in stars. However, we still lack a detailed picture of stellar burning and many questions remain such as: where is the upper mass-gap in the black-hole mass distribution?, what is the fate of stars that are initially 8-10 solar masses?, and how precisely can neutron-capture yields constrain stellar burning conditions?. Meanwhile, several devices and facilities have recently been developed that are dedicated to measuring nuclear reactions at or near the energies relevant to stars and stellar explosions. I led a whitepaper on the topic of direct nuclear reaction measurements for stellar burning, coordinating the efforts of researchers across the International Research Network for Nuclear Astrophysics. The full work, published in Journal of Physics G as a Major Report, can be accessed here. This work was featured on IOP Publishing's Physics World Weekly podcast in an interview with me.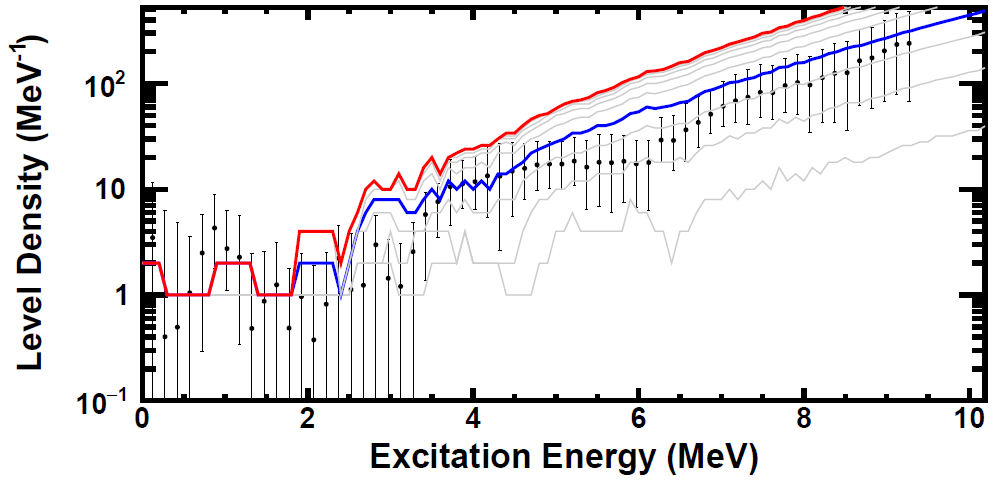
Constraining Nuclear Level Densities for Insight into Ultradense Matter
X-ray bursts on the surfaces of accreting neutron stars provide a unique window onto the nature of matter at ultrahigh densities. One avenue for understanding dense matter properties could be X-ray burst model-observation comparisons. However, uncertain nuclear physics inputs limit the viability of this approach. A particularly key reaction is proton-capture gamma-emission, (p,γ), on the nucleus copper-59. Depending on the strength of this reaction, energy generation during the X-ray burst may briefly stall, or not, impacting the shape of the burst's X-ray emission over time known as the light curve. As this nuclear reaction cannot presently be measured directly in the laboratory due to the short lifetime of copper-59, theoretical calculations are required. A statistical approach known as the Hauser-Feshbach formalism has generally been used for this reaction. However, this assumes a substantial number of nuclear levels are available to be populated when a proton fuses with copper-59 to make zinc-60. Graduate research group member Doug Soltesz led the first measurement of the zinc-60 nuclear level density. The results show that the level density is on the boundary of Hauser-Feshbach validity, meaning that measurements of the quantum mechanical spin of the relevant states, or just the spin distribution, is now necessary. There is also an interesting plateau in the nuclear level density in the nuclear excitation energy region of interest, contrary to the exponential increase expected from nuclear theory. This is the second observation of such a plateau, which is not presently understood theoretically. The full work, published in Physical Review C, can be accessed here.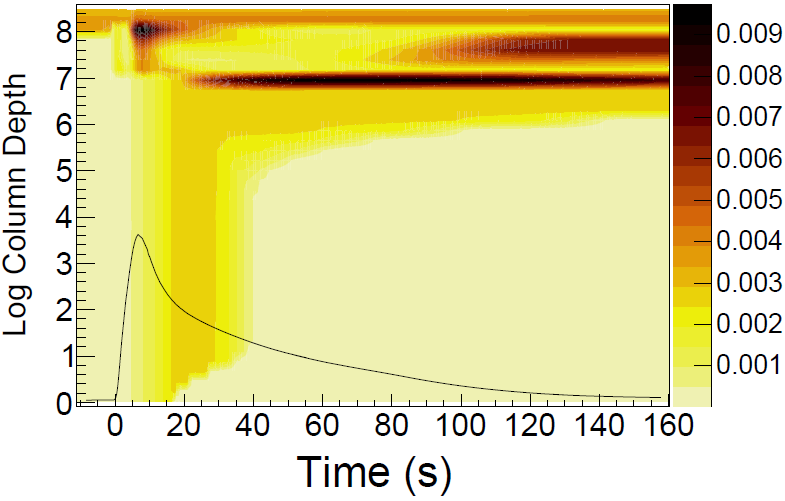
How It's Made: Urca Nuclide Production in X-ray Bursts
Neutron stars accreting fuel onto their surface from a donor star can host a number of astronomical observables, which are in turn interpreted to learn something about ultradense matter. These observables often depend on the thermal structure of the neutron star's outer layers, known as the atmosphere, ocean, and crust. Nuclear reactions in the envelope modify the thermal structure, including a neutrino emitting process known as urca cooling. Urca cooling nuclides form heat-sink layers in the accreted crust, which can modify astronomical observables. Therefore, it is important to determine the strength of urca cooling in the crust, which is related to the quantity of these nuclides. Many urca cooling nuclides are ultimately produced in the accreted atmosphere in X-ray bursts, but the details of the production process had not yet been studied. Undergraduate research group member Grant Merz led a study to detail urca nuclide production in X-ray bursts, finding the conditions of interest are different than those of interest for the X-ray burst light curve. These results will impact the details of future nuclear astrophysics experiments aimed toward studying accreting neutron star envelopes.. The full work, published in the Monthly Notices of the Royal Astronomical Society, can be accessed here.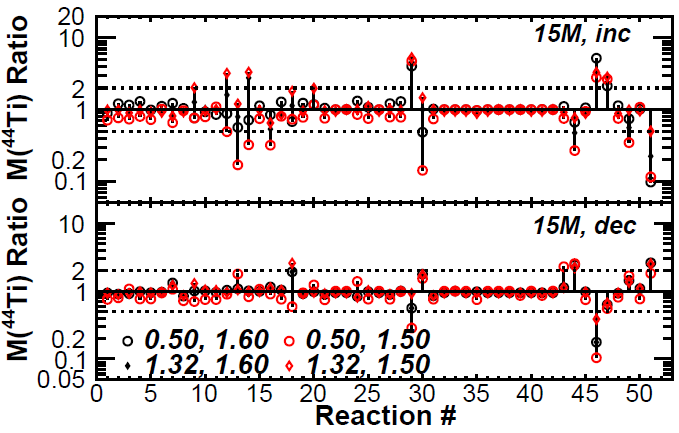
In for a Shock: 44Ti Production Sensitivity in Core Collapse Supernovae
When a star roughly ten times the mass of our sun uses up its core nuclear fuel, a catastrophic failure occurs, resulting in a rapid collapse of the stellar core. A core collapse supernova can then ensue, consisting of a brilliant flash of light and neutrinos, coinciding with nucleosynthesis of a variety of chemical elements. How the supernova is powered and how matter behaves at these extreme temperatures and densities are poorly known. The production of 44Ti (observed by γ-ray telescopes) in the shockwave that follows core collapse can offer some clues. However, 44Ti production in model calculations is known to be sensitive to nuclear reaction rates. Ohio University graduate student Shiv Subedi led the first study using one-dimensional stellar models to determine the sensitivy of 44Ti production in shock-driven nucleosynthesis to nuclear reaction rate variations for a large number of reaction rates and a variety of astrophysical conditions. The results identify several key nuclear reactions that will be the target of future nuclear physics experiments. Interestingly, the results also indicate that sensitivity study results from previous studies using pre-defined temperature and density trajectories are likely incomplete, motivating future larger scale 1D sensitivity studies. The full work, published in the Astrophysical Journal, can be accessed here.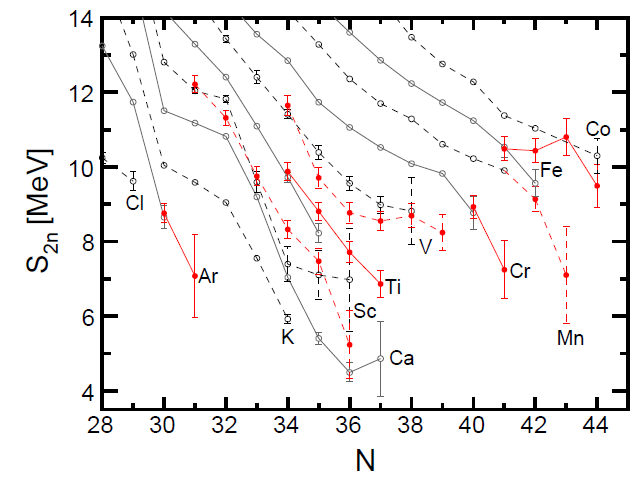
Linking the Structure of Nuclei and Neutron Stars
While neutron stars are not giant atomic nuclei, the structure of a neutron star is intimately linked to the structure of atomic nuclei. This is particularly the case for neutron stars accreting material from a binary companion, as the outer neutron star layers are comprised of terrestrially-accessible nuclei that modify the local thermal structure. We expanded the known nuclear mass surface for titanium through iron, obtaining 8 never-before-measured nuclear masses. Our results pinpoint where the magic neutron numbers 34 and 40 disappear. When considered in the context of accreting neutron stars, these new masses lead to a significantly cooler accreted neutron star crust. The full work, published as a Rapid Communication in Physical Review C, can be accessed here. A conference proceedings that places this work in context to nuclear mass measurements on exotic nuclides can be accessed here.
Sultana Awarded Master's Degree
Congratulations are in order for Irin Sultana, who recently defended her Master's Thesis, entitled "Resolving the Resonance Conflict in the 18Ne(α,p) Reaction Rate". Irin's work involved performing and analyzing a measurement of 20Ne(3He,n) to identify excited states in 22Mg of astrophysical relevance for the 18Ne(α,p) reaction rate. In particular, measurements are conflicted as to whether a spin-1 state at 8.99MeV excitation energy exists. If present, this would likely significantly alter the temperature at which Type-I X-ray bursts ignite on accreting neutron stars. Irin's measurements demonstrated that a spin-1 state is not present, indeed finding no evidence for a state of any spin at that energy. Irin will be continuing her successful career in nuclear astrophysics research at Central Michigan University, where she will join the PhD program. A copy of Irin's M.S. thesis can be accessed here.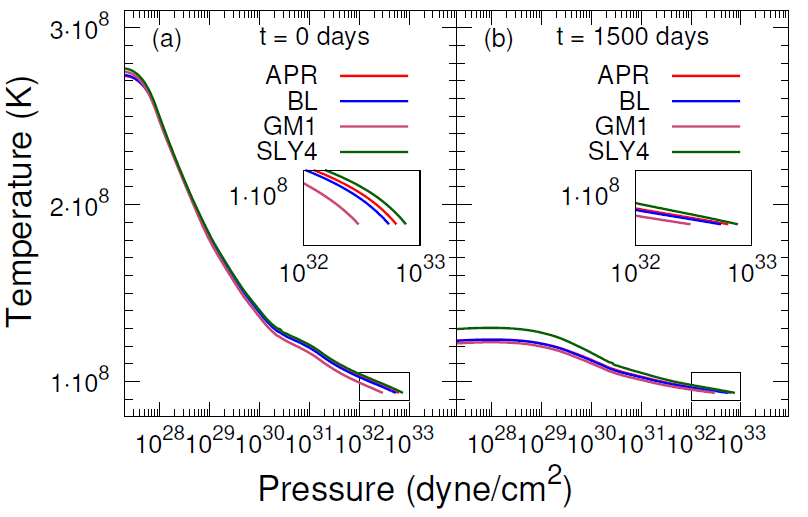
The Dense Matter Equation of State Is Important … Just Not for This Reason
The dense matter equation of state is required to describe matter at ultrahigh densities. Its elucidation is one of the primary goals of nuclear astrophysics. Neutron stars cooling off after siphoning gas from a binary companion for months or longer, so called cooling transients, are one available probe to constrain the dense matter equation of state. Models calculating the cooling of the crust (the lattice of ions making up the outer kilometer of the neutron star) can be compared with cooling transient observations for such constraints. However, one lurking issue was that the pressure (depth) at which the crust transitioned to the core (the uniform, possibly exotic, matter comprising the bulk of the neutron star) is linked to the dense matter equation of state and was thought to influence model results for crust cooling. This would severely complicate model-observation comparisons for cooling transient systems. In this work, led by Ohio University graduate student Sudhanva Lalit, we show that the crust-core transition pressure does not significantly alter model calculation results for cooling transients, thereby simplifying the associated model-observation comparisons. The full work, published in the the Astrophysical Journal, can be accessed here. Model calculation details can be accessed here.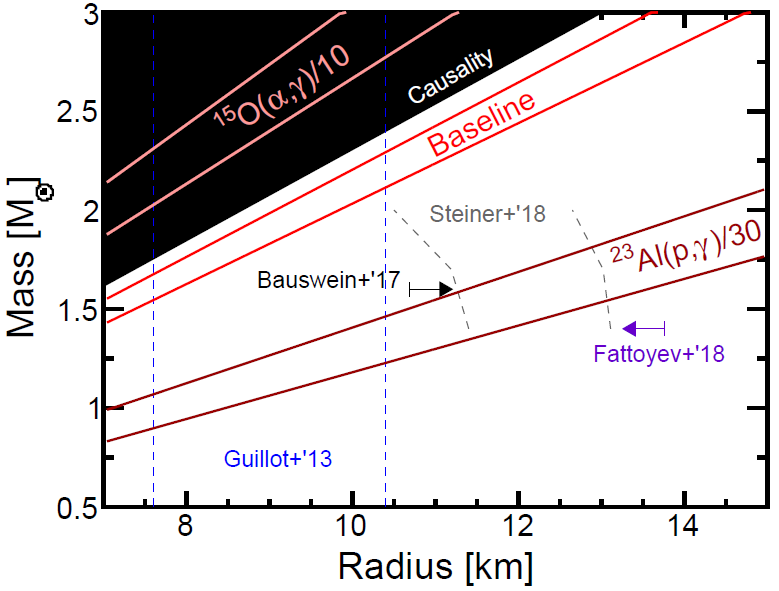
Nuclear Reaction Rates Prioritized to Constrain Ultradense Matter
Neutron stars are the ultradense remnants of massive stellar explosions and the densest objects in the universe (where density has any meaning). For neutron stars with an ordinary star like our sun located less than an earth-to-sun distance away, a variety of observable phenomena can occur which can be used to constrain properties of the underlying dense matter. In this work, the first publication for undergraduate group members Grant Merz and Sophia Medvid, we highlight key nuclear physics uncertainties that limit one approach to constrain neutron star compactness. Using numerical calculations to reproduce the light output of X-ray bursts, nuclear-powered explosions on the neutron star surface, we show that the neutron star mass-radius ratio can be extracted. However, we show that the fidelity of these constraints is strongly limited by six nuclear reaction rates. This work opens-up the possiblity for a new mass-radius constraint for accreting neutron stars and prioritizes future laboratory experiments. The full work, published in the the Astrophysical Journal, can be accessed here. Model calculation details and results can be accessed here.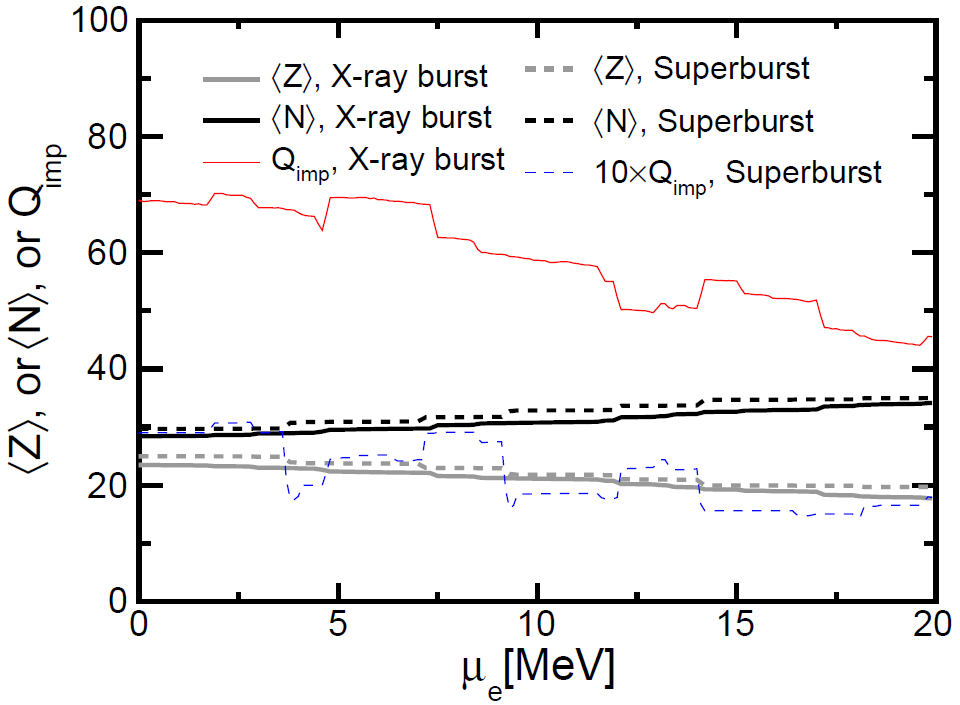
Nuclear Physics of Neutron Star Outer Layers in a Nutshell
Neutron stars, city-sized objects that weigh as much as the sun, host some of the most extreme conditions known to exist in nature. Studies of these objects ultimately aim to provide insight into the behavior of matter at densities near that of an atomic nucleus and temperatures low enough for quantum phenomena to manifest themselves. Neutron stars host a rich variety of nuclear processes, which shape their thermal and compositional structure and are especially prominent in the outer layers (often known as the atmosphere, ocean, and crust). In an invited review, we summarize the state of knowledge of nuclear physics of the outer layers of neutron stars, approaching the subject from the perspectives of observational astronomy, astrophysics theory, nuclear experiment, and nuclear theory. In this sense, this article is a one-stop-shop for practitioners looking to learn more about related research in other subfields and for students beginning to enter the exciting field of neutron star nuclear physics research. The full work, published in Journal of Physics G, can be accessed here. I gave a short seminar series in the same spirit, which can be found here.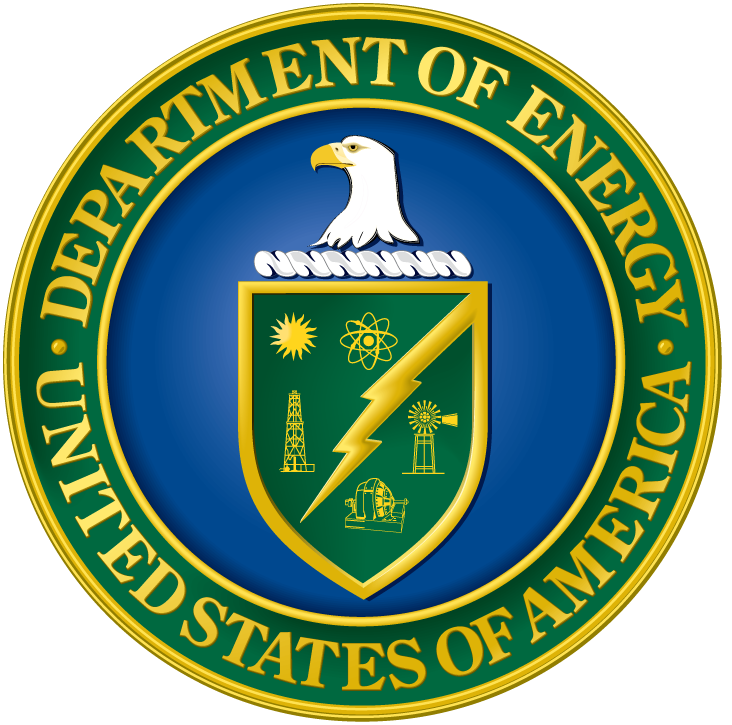
Meisel Recieves DOE Early Career Award
Research group leader Zach Meisel recieved one of the 2018 Department of Energy Office of Science Early Career Awards. This selective award "supports the development of individual research programs of outstanding scientists early in their careers" who "have the potential to develop new scientific ideas, promote them, and convince their peers to pursue them as new directions." This award will support innovative research employing nuclear physics experiments using stable and radioactive ion beams coupled with state-of-the-art astrophysics model calculations of phenomena occuring in the outer layers of accreting neutron stars. Ultimately this research will improve our understanding of how matter behaves at the highest densities observed in nature. You can read the full story in the Ohio University Compass newsletter here or the Facility for Rare Isotope Beams newsletter here and find the Public Abstract for the funded research here.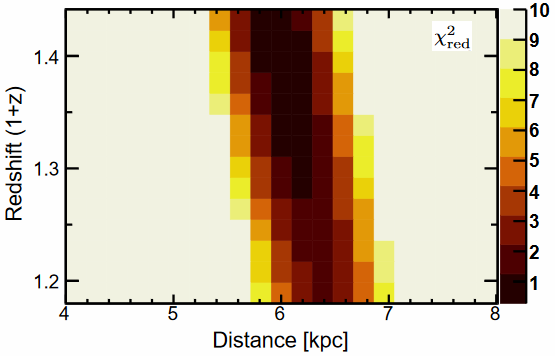
Constraining Nuclear Physics with Astrophysics: Reconstruction of the Clock Burster
Type-I X-ray bursts are thermonuclear explosions on a neutron star surface powered by hydrogen and helium burning. The "textbook" example is the so-called clock burster GS 1826-24, which has displayed regular bursting behavior that resembles results obtained from astrophysics model calculations. We consistentely modeled the light curve of three bursting epochs of GS 1826-24 for the first time. This work resulted in improved astrophysical constraints on this source and demonstrated that such light curve reconstruction may be able to place limits on nuclear reaction rates which are presently out of reach from laboratory experiments. The full work, published in the Astrophysical Journal, can be accessed here. Model calculation details and results can be accessed here.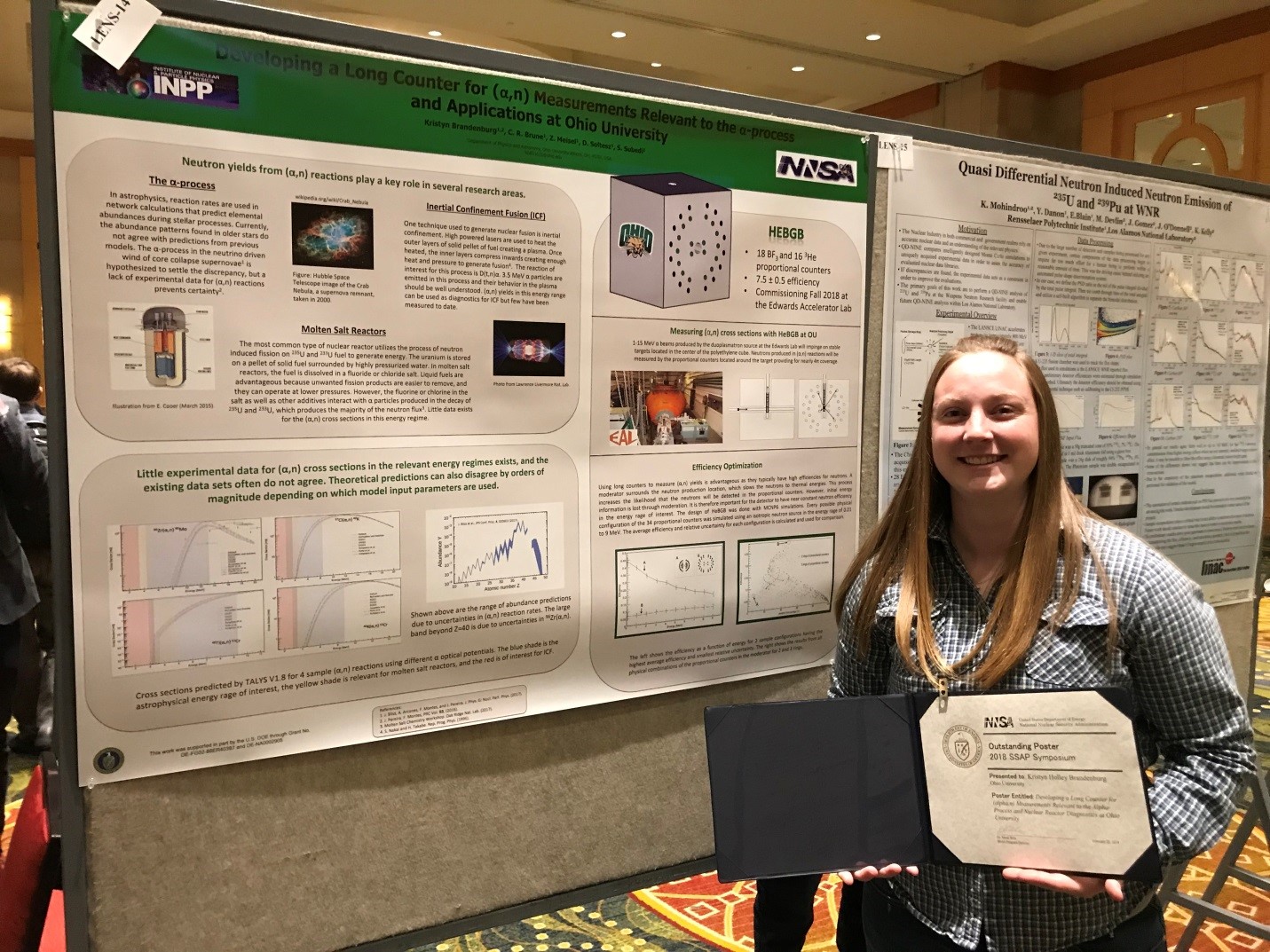
Brandenburg Recognized at SSAP Symposium
Research group member Kristyn Brandenburg took home a "best poster" prize at the National Nuclear Security Administration Stewardship Science Academic Program (NNSA SSAP) 2018 Symposium. Kristyn presented her work Developing a Long Counter for (α,n) Measurements Relevant to the α-process and Nuclear Reactor Diagnostics at Ohio University. You can read more about this story from the NNSA Stockpile Stewardship Quarterly here, or from the Ohio University College of Arts and Sciences Forum here.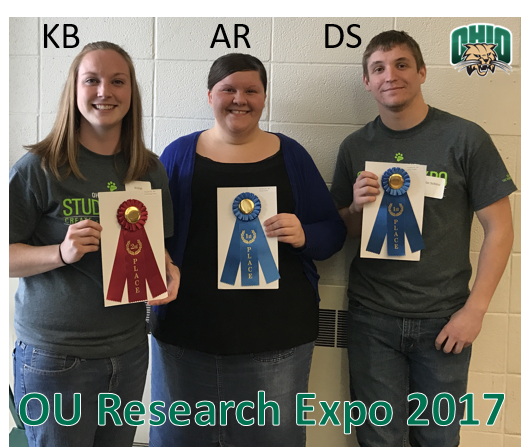
OU Expo 2017: Clean Sweep!
Research group members Kristyn Brandenburg and Doug Soltesz won poster presentation awards at the 2017 Ohio University Student Research Expo. Kristyn presented her work on the development of a neutron long-counter for measuring (α,n) cross sections. Doug presented his work on the development of a silicon detector array for detecting charged-particle emission from compound nuclear states populated via (3He,n) two-proton transfer measurements. The full story, from the College of Arts and Sciences at Ohio University, can be accessed here.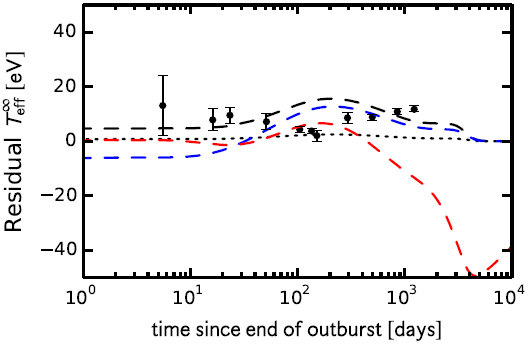
Going Back in Time with Crust Coolers
Neutron stars often siphon hydrogen- and helium-rich material from a companion star, leading to a host of nuclear reactions near the neutron-star surface and associated observable phenomena. So-called quasi-persistent transients, neutron stars that cool after a long outburst of accretion ceases, can be used to infer the thermal and compositional structure of the neutron star's outer layers. However, Urca cooling, neutrino emission from e-/β--decay cycles, has the potential to substantially impact the neutron star thermal structure. We included the impact of Urca cooling at realistic cooling strengths in models of quasi-persistent transients, shwoing it can impact the quasi-persistent transient light curve during quiesence. We use this effect to demonstrate that nuclear burning on the neutron star surface from centuries to millenia in the past can be constrained. We also identify nuclear physics uncertainties that must be reduced to improve the bygone surface nucleosynthesis constraints provided by our new technique. The full work, published in the Astrophysical Journal, can be accessed here.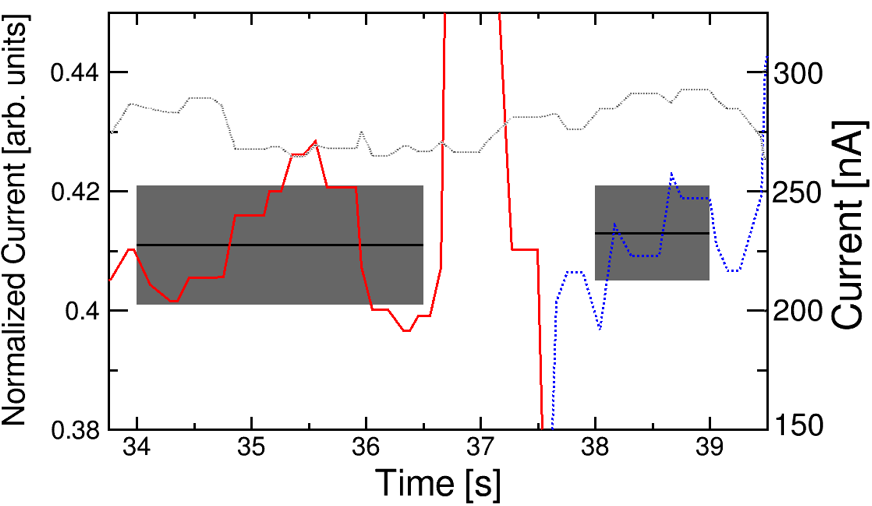
St. George Put through Its Paces
One of the most commonly occuring nuclear reaction types in stable and explosive stellar processes is the α-capture, γ-emission, (α,γ), reaction. These so-called radiative helium-burning reactions are notoriously diffuclt to measure directly with traditional techniques employing an α beam and γ-ray detector, since γ-rays of interest are often hidden due to prodigious background radiation. The St. George recoil separator was designed to work around this difficulty by instead measuring the nuclear recoil produced by (α,γ) reactions. We recently completed the crucial first test of St. George to make sure it could accept the full range of energies expected for nuclear recoils produced by (α,γ) reactions of interest for astrophysical helium-burning processes. The full work, published in Nuclear Instruments and Methods A, can be accessed here.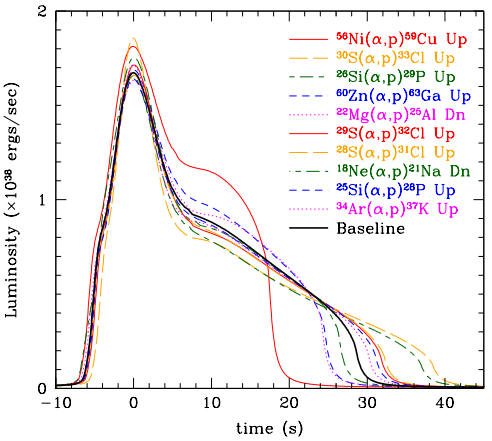
Search Narrowed for Important Rp-process Reactions
Thermonuclear x-ray bursts, one of the most commonly occuring thermonuclear explosions in the universe, have been linked to hydrogen and helium burning reactions on neutron star surfaces for roughly four decades. Though it is understood that the rapid proton-capture (rp)-process powers the bursts, systematic studies identifying the most important nuclear reaction rates have been virtually absent. In fact, to-date only one study has examined the sensitivity of the rp-process reaction network to variations in thermonuclear reaction rates. To remedy this issue, we performed the first nuclear reaction sensitivity study of the rp-process which took into account the feedback from nuclear reactions on the environment temperature. We found several reactions which affect the light output and nuclear reaction products from x-ray bursts and quantified their impacts. The full work, published in the Astrophysical Journal, can be accessed here.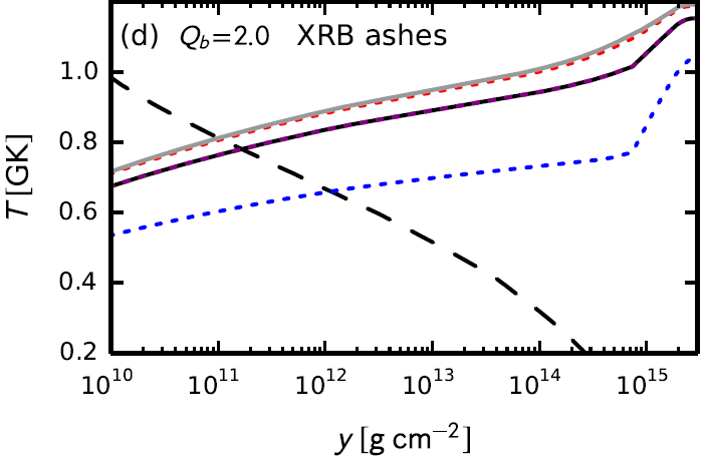
Thermostats Ubiquitous Near Neutron Star Surface
The possible existence of urca pairs, nuclides which cool dense environments via ν emission from e--capture/β--decay cycling, in the crust of accreting neutron stars was recently identified. We expanded upon that recent discovery by completing the census of all possible urca cooling nuclides throughout the neutron star ocean and crust, incorporating more realistic nuclear physics assumptions than had been made previously. We also assessed the impact of plausible urca cooling strenghts on x-ray superbursts and found a robust increase in the depth of carbon ignition (which is thought to trigger superbursts).The full work, published in the Astrophysical Journal, can be accessed here.
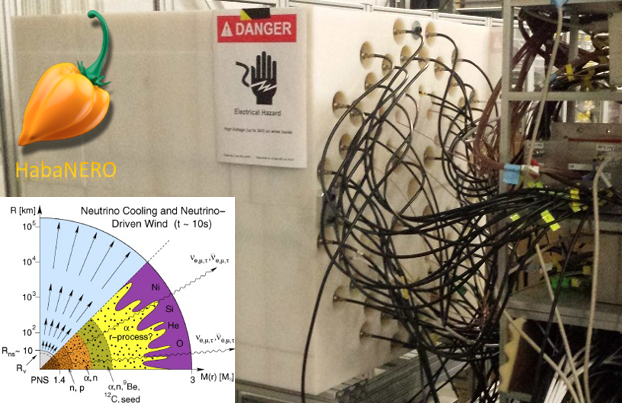
Investigation of the Origin of Zn to Sn Heats Up with HABANERO
In the past decade, it has become apparent the elements from roughly zinc (Zn) to tin (Sn) are likely not made in the rapid neutron-capture process, as was previously thought. One favored creation site is the neutron-rich ν-driven winds of core-collapse supernovae, primarily via a sequence of (α,n) reactions. However, no (α,n) reaction cross sections on neutron-rich nuclides have been measured to-date due to experimental challenges. We recently developed the HABANERO neutron detector, which will enable us to measure many of the important (α,n) reactions at astrophysically relevant energies for the first time. We have now completed our first commissioning study of HABANERO at the Edwards Accelerator Laboratory at Ohio University, demonstrating its neutron-dection capabilities.The full story, from the College of Arts and Sciences at Ohio University, can be accessed here.
Update: We completed the first (α,n) reaction cross section measurement on a neutron-rich nuclide July 2016 at the National Superconducting Cyclotron Laboratory. Analysis is ongoing.
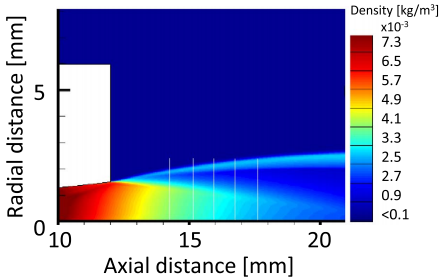
Supersonic Gas-jet Target Optimized with CFD
Supersonic gas-jet targets play an important role in nuclear astrophysics studies by providing point-like nuclear reaction targets of gaseous species. The HIPPO gas-jet will provide the helium target for (α,γ) reaction cross section measurements with the St. George recoil separator at the University of Notre Dame's Nuclear Science Laboratory. The fluid dynamics properties of HIPPO were recently investigated with computational fluid dynamics (CFD) simulations and compared to data. This study marks the first step toward optimizing the performance of HIPPO and future gas-jets as nuclear reaction targets for nuclear astrophysics studies.The full work, published in Nuclear Instruments and Methods A, can be accessed here.
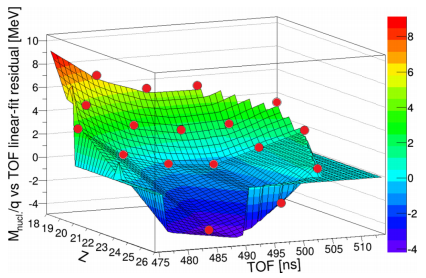
Time-trial Expands Known Nuclear Mass Surface
Nuclear masses provide key insight into the evolution of nuclear structure for highly exotic nuclides, as well as crucial input into astrophysics model calculations. Mass determinations of the most exotic nuclides accessible in the laboratory are hindered due to their low-statistics and short half-lives. We recently overcame these challenges for neutron-rich isotopes of argon through iron via time-of-flight (TOF) mass measurements performed at the National Superconducting Cyclotron Laboratory at Michigan State University. By obtaining a calibrated mass-TOF relationship, we determined the masses of 18 nuclides, 7 of which were measured for the first time.The full work, published in Physical Review C, can be accessed here.
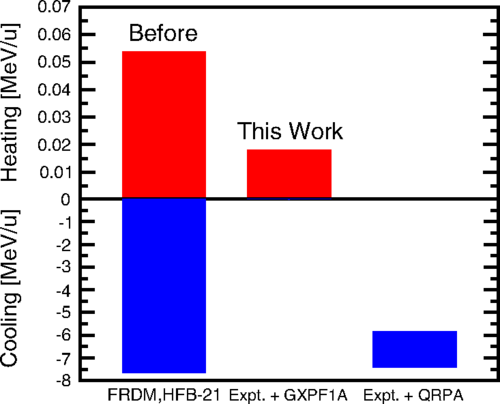
Neutron Star Crusts Not So Hot (or Cold)
Recent calculations showed that urca cooling, ν-emission from e-/β--decay cycling, may be present in the crusts of accreting neutron stars and that e--capture into 56Sc may be the strongest source of cooling. However, whether strong heating or cooling occurred depended on the unknown mass of 56Sc and the nuclear structure of its e--capture daughter 56Ca. We resolved this mystery by measuring the mass of 56Sc for the first time, using the time-of-flight mass measurement technique. When supplemented with new nuclear shell-model calculations of 56Ca's structure and implemented in a state-of-the-art neutron star crust composition evolution calculation, we found neither strong heating nor cooling. This result means that the abundance of other urca cooling nuclides, which were previously thought to have weaker cooling strengths, now must be determined to higher precision.The full work, published in Physical Review Letters, can be accessed here.
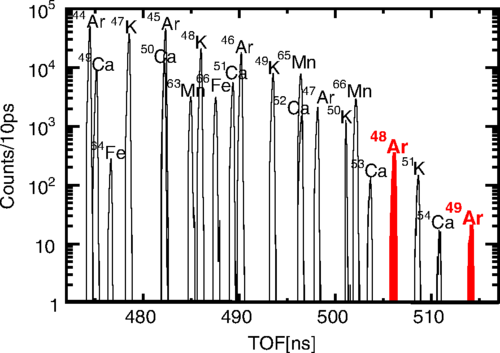
Mind the (N=28) Gap
For roughly 70 years nuclides have been known to exhibit an enhanced stability to transmutation when they possess special, so called "magic", numbers of nucleons (neutrons and protons). However, it has been shown that magic numbers disappear (and new ones can take their place) for increasingly exotic nuclides. Until now, the lower limit for the number a protons (Z) a nucleus required for it to display N=28 magicity was not known. We determined this honor belongs to argon (Z=18) using time-of-flight mass measurement.The full work, published in Physical Review Letters, can be accessed here.
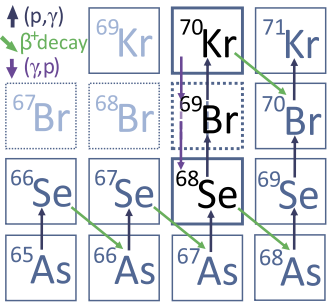
68Se Pumps the Breaks on the rp-Process
Type-I X-ray bursts are thermonuclear explosions occuring on the surfaces of accreting neutron stars. Of the thousands of possible nuclear reactions, a handful are thought to play a dominant role in the rate at which the burning of nuclear fuel proceeds, and therefore on the burst light output and burning ashes. Waiting-point nuclides play such a role in the rp-process, where burning of fuel is slow and the process stalls until the waiting-point nuclide has decayed away. Recently it was an open question as to what extent 68Se stalled the rp-process. By determining the mass difference between 68Se and its proton-capture daughter 69Br in a β+-delayed proton-emission measurement at the National Superconducting Cyclotron Laboratory at Michigan State University, we found 68Se substantially stalls the rp-process, quenching the light output of x-ray bursts at late times.The full work, published in Physics Letters B, can be accessed here.
The instrumentation paper corresponding to the new analysis technique developed for this work, published in Nuclear Instruments and Methods A, can be accessed here.